Extreme events
Our group investigates a wide array of weather and climate extremes across the globe, and on various temporal scales: from short timescale heavy precipitation events in desert regions (Moshe “Koko” Armon) and in eastern Europe (Ellina Agayar), to multi-day heat and cold waves (Matthias Röthlisberger), entire extremely wet, dry and hot seasons across the globe (Hanin Binder) and the physical causes and multi-year aggregation of seasonal extremes in the Arctic (Katharina Hartmuth). Overarching research goals of our work are (a) to improve our understanding of the meteorological chain of events that lead to these extremes (i.e., process understanding), (b) to evaluate global climate models, in particular models from the CESM family, regarding their ability to realistically simulate the meteorological processes leading to those extremes and (c) to evaluate how these events will change under global warming, in terms of number, severity and physical functioning. Specific examples of our research include the following:
Desert precipitation extremes:
In deserts, almost every rainfall event is an extreme event. Some events are more severe than others, triggering flash floods and other natural hazards. At the same time, such heavy precipitation events replenish water resources, fill desert lakes, and contribute to unique desert ecology that depends on rainfall occurrence. Desert inhabitants are disproportionately exposed to such natural hazards and are most vulnerable to climate change, making them more susceptible for the adverse impacts of global warming. While space-time variability of rainfall is especially high in deserts, direct observations are limited and models fail to reproduce the small-scale precipitation. Therefore, it is challenging to even determine where and when rainfall occurs, more so why it happens. To better understand what atmospheric conditions lead to heavy rainfall in deserts and how these would change with climate change, we use (a) satellite data to observe precipitation and the filling of desert lakes, (b) reanalysis data to infer the meteorological mechanisms involved, and (c) global climate models to project future changes in these mechanisms. This research is led by Koko (Moshe) Armon.
Extreme precipitation in Ukraine:
Anthropogenic climate change not only affects mean climate conditions, but changes are also expected in the temporal variability of extreme meteorological events, including precipitation. Understanding extreme precipitation events (EPEs) and their underlying dynamical processes and moisture transport patterns is essential to mitigate EPE-related risks, which pose a great threat as a trigger for landslides and floods. The genesis and spatiotemporal variability of EPEs in midlatitude regions are a consequence of complex dynamical and thermodynamical processes that occur on the synoptic and mesoscale. Within the project, we studied characteristics of EPEs in terms of the large-scale flow and moisture source conditions for Ukraine in the last 40 years. Taken together, these findings provide novel insight into large-scale characteristics of EPE in Ukraine, in a region with a quite complex orography and diverse moisture sources. The senior scientist working in this research area is Ellina Agayar.
Physical functioning of heat extremes:
Ever more intense heat extremes are a hallmark of 21th century climate change. Yet, the scientific world still has a poor understanding of what determines the magnitude of exceptionally intense heat waves, of how very intense heat waves differ from less intense ones, and of how well the responsible physical processes are simulated in state-of-the-art climate models. We developed and now use a Lagrangian method that allows quantifying the contributions of air mass advection, adiabatic warming/cooling and diabatic processes to temperature anomalies in temperature extremes and apply it to heat and cold extremes in reanalyses and climate model data. This research is led by Matthias Röthlisberger.
Seasonal extremes:
Meteorological extreme events occurring on a seasonal timescale are of high social, environmental, and economic relevance. For instance, extremely dry or cold springs can have adverse consequences for agriculture, while particularly stormy seasons can lead to substantial aggregated damage and thereby pose a challenge for the reinsurance sector. A newly developed method has been applied to ERA5 reanalyses and 1000 years of global climate simulations to identify extremely wet and dry, windy and calm, and hot and cold seasons across the globe. This allows us to systematically investigate the characteristics of extreme seasons in the present-day climate and to quantify potential changes in the charac-teristics in a future climate. For example, we aim to assess whether certain extreme seasons are associated with anomalous cyclone and anticyclone frequencies, or whether they go along with anomalous surface conditions at the beginning of the season. The senior scientist working in this research area is Hanin Binder.
Arctic extreme seasons:
The Arctic is strongly affected by climate change and climate models predict the transition towards a seasonally ice-free Arctic Ocean within the 21st century. At the same time, Arctic surface conditions exhibit a large variability on synoptic to interannual temporal scales. There is still a considerable lack of knowledge regarding Arctic variability and extremes on the intermediate seasonal time scale. We use a combination of reanalysis and climate model data to better understand the relative importance of internal atmospheric variability and boundary conditions for Arctic interannual variability in both present-day and future climates. This research is led by Katharina Hartmuth.
Global analysis of high-impact cyclones in ERA5 and CESM2 simulations

Sven Voigt
Extratropical cyclones, together with their attendant fronts and moist airstreams, are the predominant weather system responsible for extreme surface winds and precipitation across the majority of the extratropics. Nevertheless, confidence in geographical projections of extratropical cyclone activity is low.
This PhD project investigates extreme cyclones in terms of their impact on surface winds and precipitation. The occurrence of local surface wind and precipitation extremes is evaluated along all cyclone tracks globally within the ERA5-reanalysis dataset. As a result, each cyclone will be associated with its number of extreme impacts, which allows to identify the most extreme cyclones according to this dimension of extremeness. By analyzing local weather extremes—defined as events where mean wind speed or accumulated precipitation exceed the 99.9th percentile at a specific grid point—across timescales from 1 hour to 3 days, different types of cyclones are likely to emerge as the most extreme. For example, it is expected that short-duration surface wind extremes occur with deep and fast-moving cyclones, while extreme long-duration precipitation is more likely to be associated with stationary but only weakly intensifying cyclones. In a second step, the approach is applied to CESM large ensemble simulations to assess potential changes of the properties of extreme high-impact cyclones over the course of the twenty-first century. Focusing on the dynamics of these cyclones, case studies of the most extreme cyclones affecting a particular region in both present-day and future climates will then be performed.
Supervised by Heini Wernli and Hanin Binder.
The vertical dimension of extratropical heat extremes

Heat extremes belong to the most severe natural hazards. With global warming, their frequencies and intensities are expected to increase. Therefore, reliable projections of these events are essential. The accuracy of climate model projections of these extremes depends on a comprehensive understanding of the involved physical processes and their correct representation in climate models. Previous studies have examined surface conditions and processes focusing on temperature, precipitation, and soil moisture, as well as the upper-level flow. However, the importance of the vertical structure of the atmosphere during heat extremes has only been recently recognized, and there is only an anecdotal understanding of the entire 3-dimensional dynamical processes during heat extremes. However, the vertical structure could be insightful for answering what distinguishes the most intense heat extremes from more moderate ones.
This PhD project aims to tackle these research gaps by using reanalysis and climate model data. Therefore, the atmospheric vertical structure and its key characteristics during heat extremes will be documented in ERA5. Moreover, this project also aims to elucidate the difference between the most intense and more moderate heat extremes in the vertical profile. By quantifying the contributions of advection, adiabatic warming/cooling, and diabatic processes to the temperature anomaly profile of anticyclones within which particularly widespread and intense heat extremes occurred, the focus shifts towards anticyclone-heat extreme couplets to refine our understanding of how heat extremes develop within extratropical anticyclones. Finally, all conducted analyses will also be applied to CESM2 data to evaluate the climate model's ability to simulate these structures realistically. Addressing these research gaps aids in gaining a more comprehensive understanding of heat extremes and improving the representation of heat extremes in climate models and projections.
Supervised by Robin Noyelle and Heini Wernli
Dynamics and drivers of Arctic extreme seasons in present and future climates
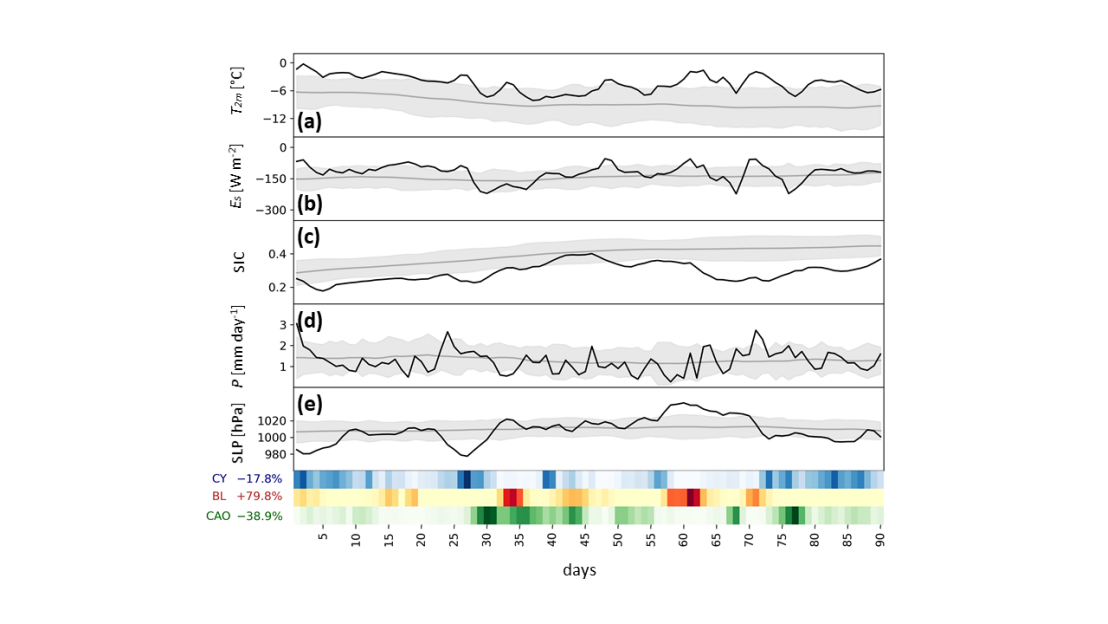
The Arctic region is expected to respond strongly to extreme events and even more to whole anomalous seasons, as it reacts particularly sensitive to climate change and thereby shows a large internal variability. Thus, extreme seasons could have a major impact on Arctic surface temperatures, formation and melting rates of sea-ice and minimum and maximum sea-ice extent. An extreme season can be defined as a 3-monthly period when specific meteorological parameters, such as surface temperature, reach extremely high or low seasonal mean values in a certain region.
The dynamical processes leading to such anomalous seasons in the Arctic as well as their possible change in a warmer climate have not yet been analysed in detail. This research project combines the detailed analysis of Arctic extreme seasons and their underlying atmospheric dynamics in the ERA5 reanalysis dataset with CESM climate model simulations. The aim is to investigate the relative importance of the different atmospheric dynamical drivers behind the sub-structure of extreme seasons in the Arctic Sea based on case studies. Further, the representation of the processes favouring extreme seasons as well as the ability to reproduce such anomalous seasons will be investigated for the CESM model. The evaluation of future climate simulations will provide information about the structure and development of future Arctic extreme seasons.
Supervised by Lukas Papritz, Maxi Böttcher and Heini Wernli
Assessing the dynamics and substructure of extreme seasons, as defined from their impact, in present day and future RCP8.5 climate
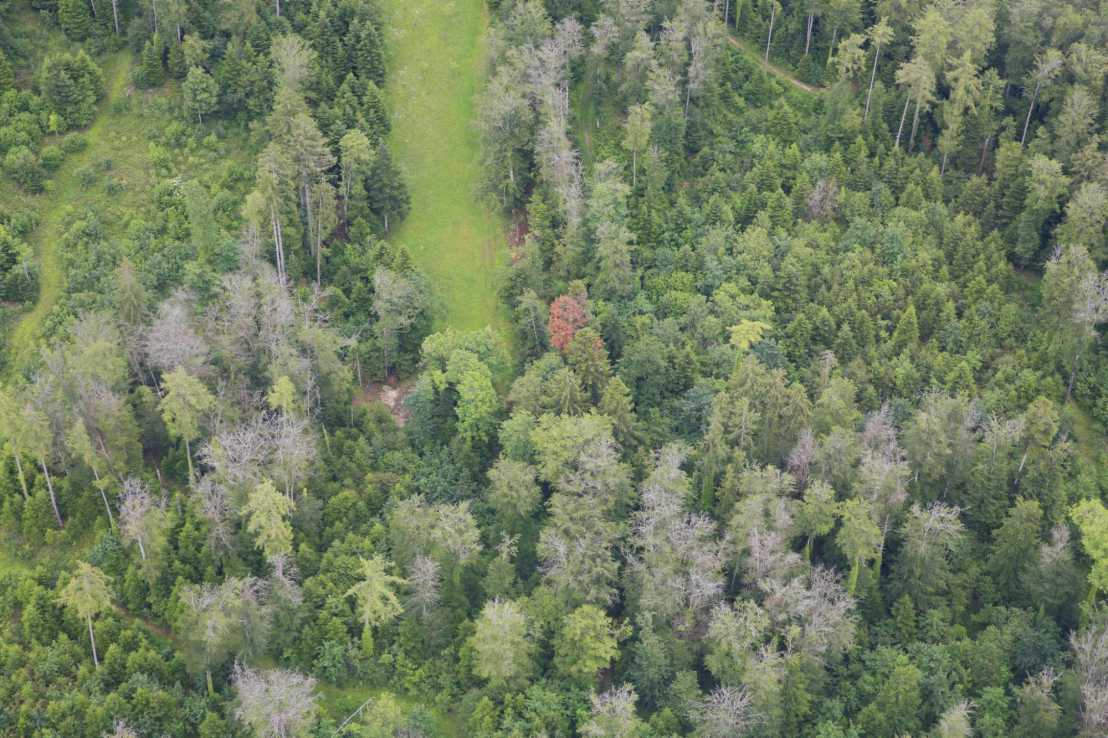
Mauro Hermann
A broad range of knowledge has been acquired on both the occurrence and impact of extreme events as well as climate trends under global warming. On the one hand, the timescale linked to extreme event studies is typically in the range of hours (strong convective precipitation) to weeks (mid-latitude heatwave). On the other hand, global warming is assessed by the evolution of average climate conditions over several decades. On the seasonal timescale, however, different dynamical processes are dictating the large-scale atmospheric conditions, which have potentially devastating effects on society, our natural environment, or the climate system itself.
The extremely dry summer 2018 in Central and Northern Europe strongly reduced the ecosystem vitality and gave rise to extensive insect outbreaks, still affecting forests in the following year. With this project we try to answer questions that emerge from such seasons: Which synoptic features foster seasonal disturbances of forests? Will such extreme impact seasons change their characteristics with climate change? Further in this thinking, the (in the low Alpine regions) non-existent winter 2019/20 can be addressed due to its impact on winter tourism, or extremely warm summers over the Greenland due to their effect on the ice sheet’s mass loss.
Supervised by Matthias Röthlisberger and Heini Wernli
Photo credit: external page WSL