Research
Climate models and their interpretation
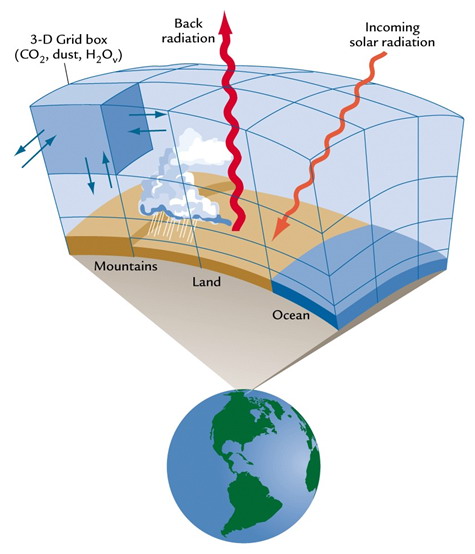
Three-dimensional global coupled climate models have an atmosphere, ocean, land and sea ice to make projections of the future climate. These models are based on a system of differential equations describing physics, fluid motions and chemistry. Institutions around the world pour their resources into the development and running of these models. The output is then interpreted to make statements and inferences about the climate system. Nevertheless, the difficulty in interpreting the results arises from models showing very different response to the same forcing. This can be because of the chaotic nature of the climate system as well as fundamental differences between how those models are constructed.
Despite the fact that models are getting internally more complex and better in simulating present day climate, they still disagree as much about the future as they did 10 years ago. This gives rise to a question, can we trust some models more than others for climate projections a century ahead? We do not have observations of the future climate to verify the models. Therefore, one has to identify some other metric to pick models that are trustworthy. Hence efforts are being made to identify what are the relevant mechanisms in present day that allow us to have more accurate projections of the future climate.
Atmospheric variability
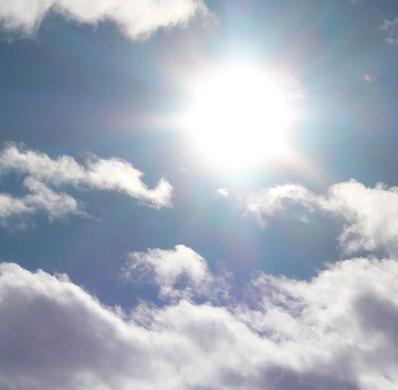
The atmosphere is a thin film of gas surrounding the Earth and is set into motion mainly due to differential heating of the earth’s surface by the sun. There are many processes occurring within the atmosphere that interact on different timescales, which makes its behavior highly unpredictable. Owing to this chaos individual weather events occur randomly, but on average, the location and certain features of these events are stable over longer periods of time. This implies that the atmosphere has preferred patterns of circulation that governs the regional climate. One of the most prominent patterns of the atmospheric variability is the North Atlantic Oscillation, often referred as NAO. It can be seen by observing fluctuations in the difference of atmospheric sea level pressure between Iceland and the Azores. This pressure difference reflects the strength and location of westerly winds in the mid latitudes.
In the climate change context, the atmospheric variability can mask the warming signal for decades on regional on even global scales in relation to the ocean. Hence it is possible that some regions will experience cooling while on average the Earth is warming. This poses huge limitations for projections of regional climate where models tend to disagree between each other more than the local warming signal driving the climate system. As a result in some parts of the world it is hard to evaluate the impacts of climate change, precluding the development of necessary adaptation and mitigation measures.
Ocean variability, circulation and response to climate change
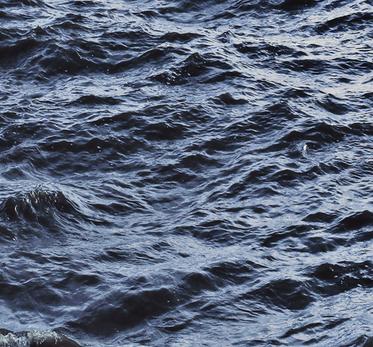
The ocean is a crucial component of the climate system and is known to influence Earth's weather and climate. The ocean is important because of it's ability to store and transport large amounts of heat, freshwater, and carbon, and also exchanges these properties with the atmosphere. In the climate change context, the ocean acts as the largest heat reservoir. Because of the long timescales in the ocean, there is currently an imbalance in the climate system. The ocean takes up heat and therefore ‘hides’ part of the warming signal at the surface. Understanding how the ocean responds to climate change, both with regards to heat uptake and circulation patterns, is a crucial element in understanding the transient response to the climate forcing.
On timescales of up to a few years the El Niño Southern Oscillation, or ENSO, is the main mode of variability in the tropical Pacific. ENSO represents temperature variations in the tropical Pacific varying between colder and warmer than normal states that are driven by changes in the atmospheric circulation. These changes again affect weather the world, even far away from the tropical Pacific. On longer timescales, both the Pacific and the Atlantic play a role in modulating the global temperatures. For these changes both the interaction with the atmosphere and changes in the ocean circulation plays a role.
Extreme events
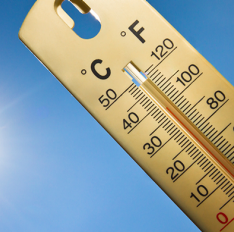
Extreme events arise due to chaotic nature of the climate system through complex interplay between its components. These are out of the ordinary events that may have significant ecological, economic and societal impacts across the globe. Heat waves, for instance, can be devastating in particular for the communities that are not used to coping with them. During the hot summer of 2003 tens of thousands of deaths all over the Europe were attributed to such an event. Climate models are commonly used to understand how these events develop. A combination of atmospheric high-pressure systems, soil moisture and land interactions have been identified as the main drivers in the development of heat waves. For other types of extremes such as precipitation, climate models have greater difficulties mainly because of small-scale processes that models are not able to simulate accurately and spatial and temporal structure of the extreme precipitation field.
The importance of the impacts is undeniable hence how the nature of these extreme events will change with climatic change is subject of intensive research. Changes in their frequency and intensity will determine the adaptation strategies that we would have to undertake. However, due to rare occurrence of these events, the confidence in their projections using climate models is usually low. Climate models themselves are not perfect and trustworthy real world observations are not available everywhere across the globe to evaluate the models. Projections disagree on the local changes, nevertheless aggregating changes over larger regions can lead to an increased consistency of the projections.