Liquid-liquid phase separation within aerosol particles
Background
The organic aerosol fraction consists of a complex mixture of partly oxidized organic compounds from natural and anthropogenic sources. We showed in external page Marcolli et al. (ACP, 2004) that the thermodynamically stable state of such complex mixtures is a liquid or amorphous solid.
If significant amounts of non-polar compounds from e.g. primary anthropogenic emissions are mixed together with oxygenated organics, liquid–liquid phase separation (LLPS) may occur. The presence of inorganic salts strongly enhances the tendency for LLPS because the aqueous solubility of organic substances decreases when electrolytes are added (salting-out effect).
The phase state of aerosol particles is relevant for different properties of aerosols and processes occurring in or on aerosol particles. Thus, LLPS influences heterogeneous and multiphase chemistry, gas-particle partitioning of semivolatile species and the activation of aerosol particles to cloud droplets.
Experiments
We started to investigate LLPS in bulk experiments with mixtures of PEG-400, ammonium sulfate (AS), and water. We determined the water activity of these mixtures with a commercial dew point water activity meter and detected phase separation optically. While PEG-400 and water are miscible at any molar ratio, we observed LLPS in the presence of AS when the relative humidity (RH) fell below about 90 %. We then decided to investigate this system in the electrodynamic balance, however, we could not find direct evidence of LLPS: the fringe pattern in the phase function and the glare spots of the elastically scattered light were consistent with spherical symmetry (external page Marcolli and Krieger, JPC A, 2006). Currently, Nir Bluvshtein is looking into this in more detail.
Therefore, we decided to investigate LLPS in micrometer-sized droplets deposited on a hydrophobic substrate in a cell with controlled RH. With this setup Gabriela Ciobanu was able to directly observe LLPS optically while she decreased RH in the cell. Moreover, she determined the composition of the phases by micro-Raman spectroscopy. The figure below is taken from external page Ciobanu et al. (JPC A, 2009). It shows LLPS of a mixture of PEG-400 and AS with mass ratio OIR=1:1 by spinodal decomposition while RH was decreasing.

Our research on LLPS was continued by Mijung Song who investigated a wide variety of organic model compounds including dicarboxylic acids (external page Song et al. ACP, 2012), polyols, oxidized aromatic compounds, and multifunctional compounds (external page Song et al., GRL. 2012; FD 2013). In addition to core-shell morphology, she found also examples for partially engulfed morphology, e.g. in the Figure below (frames 4 – 7, taken from external page Song et al., FD 2013) for mixtures consisting of C6 and C7 dicarboxylic acids, AS, and water.

We found that LLPS always occurred for O:C < 0.56, never occurred for O:C > 0.80, and depended on the specific types and compositions of organic functional groups for 0.56 < O:C < 0.80 (external page Song et al., GRL 2012).
Thermodynamic modeling
We also modelled LLPS of the investigated systems using AIOMFAC. The figure below is taken from external page Song et al. (ACP, 2012) and shows the calculated Gibbs energy difference G between a hypothetical one-liquid-phase state (1ph.) and a two-liquid-phases state (2ph.) (color coded) for (a) the C6/AS/H2O and (b) the C7/AS/H2O system at 298 K. The computed coexistence curves are shown in white (dashed where AS is supersaturated). The solubility limit of AS (deliquescence onset) is shown as the white dashed-dotted curve. The light grey dashed lines are isolines of constant equilibrium-state water activity. They represent tie-lines within the LLPS region.
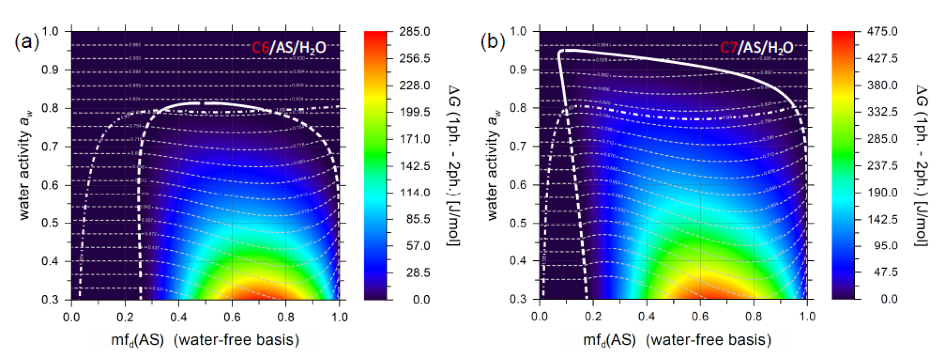