High-Resolution Climate Modeling
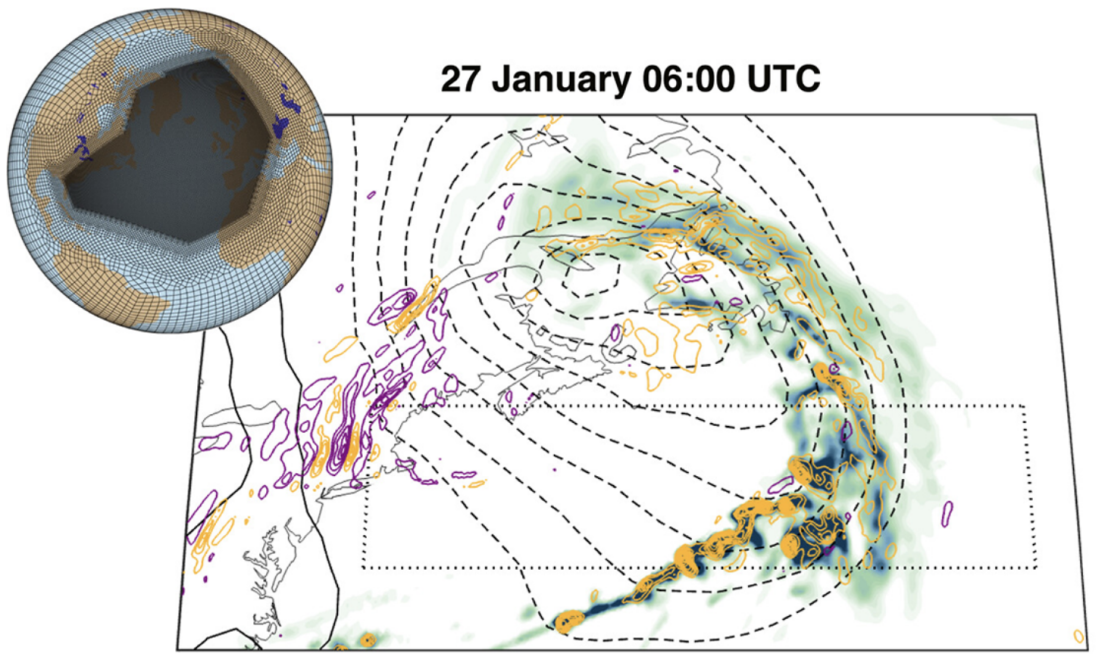
Much of the uncertainty in climate projections stems from the need to empirically parameterize processes on scales smaller than can be resolved explicitly in global climate models, such as clouds, convection, and turbulence. Recent advances in computational resources and modeling now allow simulations at higher resolutions than were previously possible, such that mesoscale processes (at horizonal scales of 10-100 km) can be represented in global models. These new high-resolution models provide an unprecedented opportunity to understand the two-way interactions between mesoscale processes, which are important in the dynamics of weather systems, and the large-scale climate system. Our research uses a hierarchical modeling approach across a range of resolutions and configurations in the CESM and ICON climate models to understand (1) the role of frontal processes in atmosphere-ocean coupling in midlatitudes and the potential for improved climate predictions from higher resolution models that resolve these processes and (2) differences in tropical climate and its response to external forcings between high-resolution configurations with resolved deep convection and coarse-resolution configurations with parameterized deep convection. Our overarching goals are both to understand the fundamental dynamics of cross-scale interactions between the mesoscale and global scale and to develop new modeling and machine learning methods to bring the added value of representing mesoscale processes into coarse-resolution global models, which are still needed to broadly investigate climate change scenarios.
Current Projects
The pattern effect on global climate with explicit versus parameterized deep convection - Clarissa Kroll, Robb Jnglin Wills
The equilibrium climate sensitivity (ECS), the warming following a doubling in CO2 concentrations, is the cornerstone upon which climate mitigation and adaption strategies are built. However, its true value is highly uncertain, primarily due to uncertainty in the cloud feedback. The atmospheric response to the "pattern effect", the spatial pattern of ocean surface warming, is a substantial source of uncertainty in this regard, as the convection- and gravity-wave-mediated propagation of temperature anomalies from near the surface in convective regions into the upper atmosphere has strong impacts on global cloud cover. The pattern effect is thus important for setting the global radiative feedback and ECS. Accurately modeling the ocean warming pattern and the atmospheric response to this warming pattern is key for accurate projections of the climate response to climate change scenarios. In this project, we compare the simulated atmospheric response to key patterns of ocean temperature anomalies in low- and high-resolution ICON simulations. Specifically, we investigate the upscale influence of the newly resolved small-scale processes on the large-scale circulation and climate in the high-resolution simulations and the corresponding influence on the simulation of the pattern effect compared to the low-resolution simulations. The pattern effect is an ideal test case for how increased resolution can improve the simulation of climate change, because it can benefit from explicitly resolved deep convection.
Finished Projects

The impact of lower stratospheric heating perturbations on atmospheric moisture and deep convection - Clarissa Kroll
Volcanic eruptions lead to heating perturbations in the tropopause and lower stratosphere, as would geoengineering relying on stratospheric aerosol injection. This has impacts on moisture transport into the stratosphere both in the form of water vapor and in the form of frozen moisture originating from deep convection. Whereas the water vapor transport is controlled by the larger-scale tropical cold-point temperatures, and therefore sufficiently described by conventional coarse-scale simulations, a realistic description of frozen moisture transport by deep convection is only achieved with km-scale simulations. We study both moisture transport pathways using a hybrid approach: For the study of water vapor entry values we rely on coarse-scale large ensemble simulations for volcanic eruptions of different magnitudes (Download Kroll et al., 2021). For the frozen moisture entry within overshooting convection, we narrow in and select one volcanic eruption magnitude to investigate with km-scale simulations. The km-scale simulations then allow us to study the effects of heating perturbations on single deep convective systems (Download Kroll et al. 2023, Download Kroll et al. 2024).
We have synthesized the findings from coarse and high resolution studies by combining them in one thermodynamically based description of the involved processes. This formulation allows a fast estimation of changes in stratospheric moisture content. The findings can be used to improve upon the currently used parameterizations of overshooting convection, estimate the impact of future eruptions, or to bring the stratospheric moisture increase, i.e. after the 2022 Hunga eruption, into context (Download Kroll and Schmidt 2024).